(All figures below are subject to copyright, and are allowed to be used only with permission from the owner.)
Anthropogenic influences on extreme precipitation events over the Pearl River Delta (PRD) region in South China with the use of a convection-resolving model
Rui ZHAO
The CMIP5 global models show that the PRD region has warmed by around 0.9 ℃ since the pre-industrial era, resulted from the increasing emission of anthropogenic greenhouse gases. As the climate warms, the atmosphere is expected to hold more moisture in line with the Clausius-Clapeyron (CC) relation, which increases the potential risks of precipitation extremes as a result. Indeed, observational results have corroborated the increasing trend of extreme rain intensity and the enhanced likelihood of such events over the PRD, which threaten our environment and society.
My research aims to quantitatively investigate the extent to which PRD extreme rainfall in different seasons can be attributed to human influences and the underlying physical mechanisms. Attribution results suggest that human activities could have contributed at least a 10% increase in daily extreme precipitation over the inland PRD. In particular, changes of rainfall in MJJAS (i.e. from May to September) are nearly CC scaling of 7% K⁻¹, whereas a super-CC increase of 12.4% K⁻¹ is found for non-MJJAS rainfall. The greater increment of non-MJJAS rainfall highlights the important role of dynamic effect on heavy precipitation, in addition to the moisture-driven thermodynamic effect.
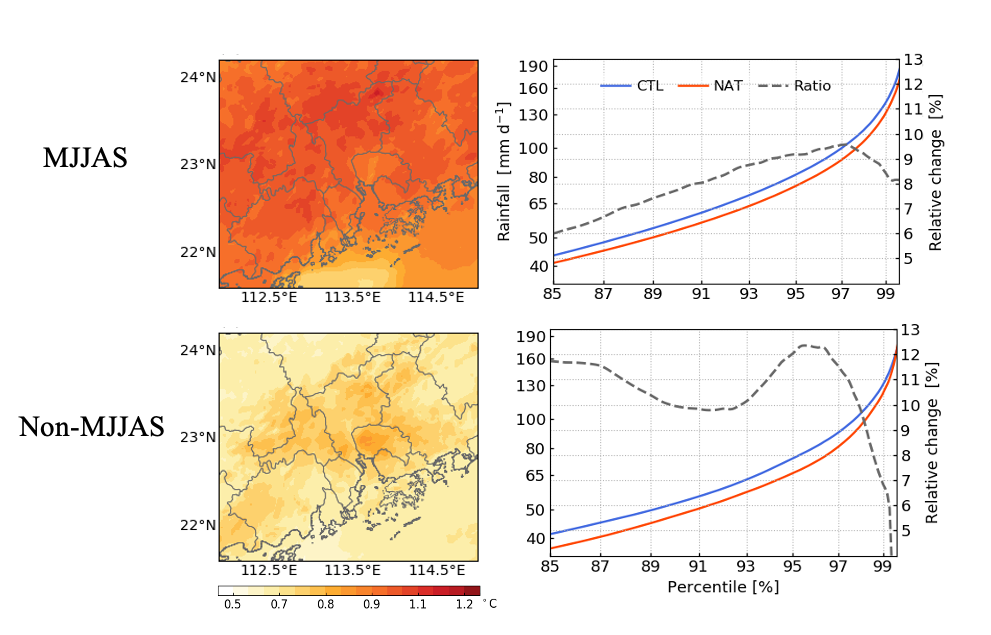
The importance of future urban development in hourly extreme rainfall projections- comparing global warming and urbanization forcing over the Pearl River Delta region
Chenxi HU
The impacts of future urban development and global warming forcing on hourly extreme rainfall over the Pearl River Delta (PRD) area have been investigated, by dynamically downscaling General Circulation Model (GCM) outputs using the Weather Research and Forecasting Model (WRF) at convection-permitting resolution, coupled with an Urban Canopy Model (UCM). Three downscaling experiments corresponding to different urban land cover (1999 and projected 2030) and climate (1951-to-2000 and 2001-to-2050 GCM simulations) were designed. Near-future climate change (up to 2050) and 1999-to-2030 urban development effects on PRD extreme precipitation were then examined. Results show that climate change and rapid urban development forcing have comparable positive effects on the intensity as well as heavy hourly rainfall probability over the PRD megacity. Global warming tends to increase heavy rainfall probability (from 40 to 60mm/hr) by about 1.3 to 1.8 times, but suppresses the frequency of light rainfall. Urban development increases urban rainfall probability within the whole range of intensity, with frequency for very heavy rainfall (> 90mm/hr) almost doubled. Overall, forcing due to rapid urban development plays an important role for projecting rainfall characteristic over the highly urbanized coastal PRD megacity, with impacts that can be comparable to global warming in the near future.
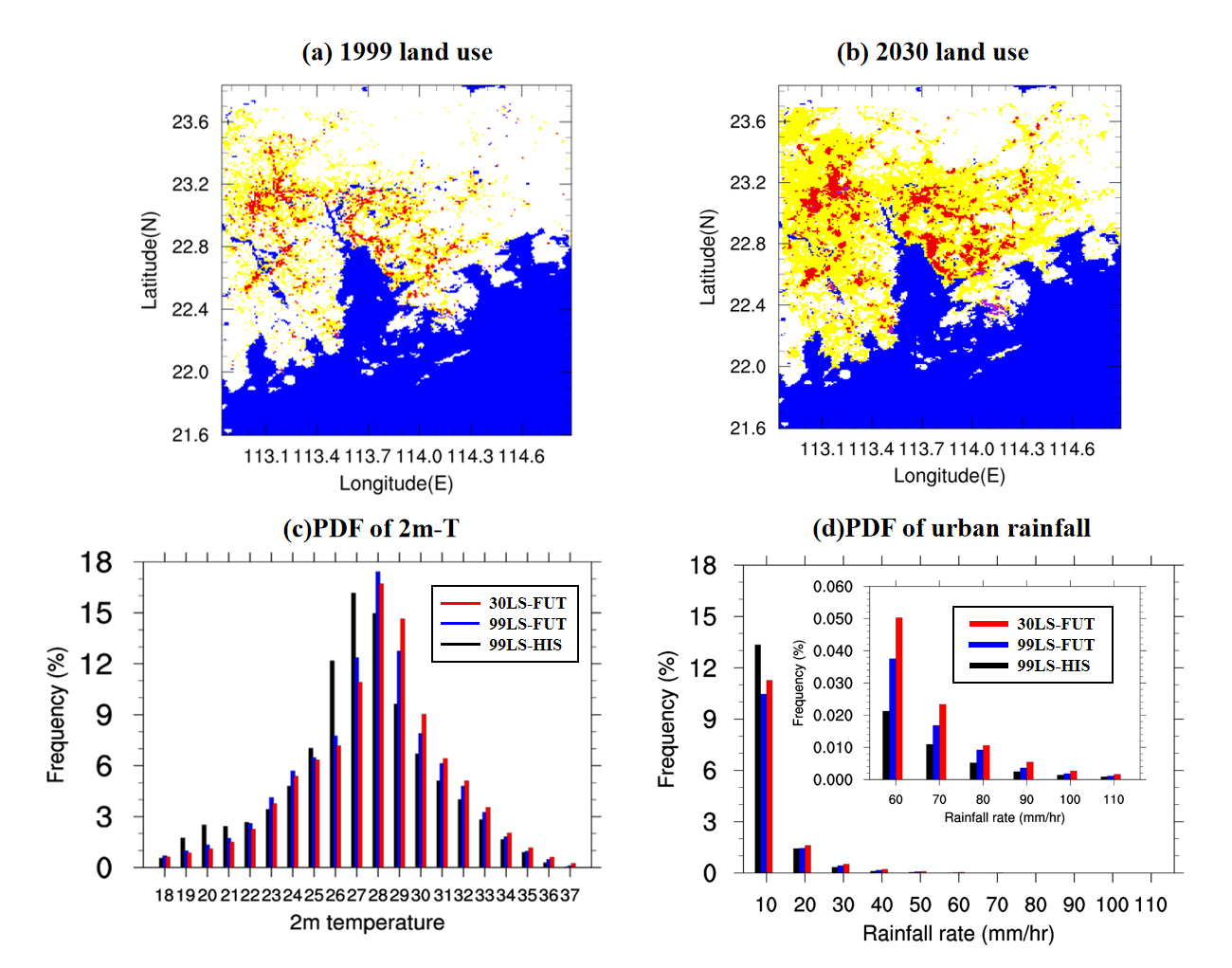
Impacts of decaying EI Niño on East Asian (EA) extreme precipitation and its diversity
Dingrui CAO
The spatial distribution of extremes shows the opposite spatial patterns during Eastern-Pacific (EP) and central-Pacific (CP) El Niño. More intense spring extremes over the southern China (SC) are observed during EP El Niño, while the situation is revise during CP El Niño.
In decay summer, intense extreme events over the south of Yangtze River (SYR) during EP El Niño and less extremes over the Mei-Yu rain band in China, Baiu in Japan, Changma in South Korea (MBC) are observed. During CP El Niño, weaker (stronger) extremes over SYR (MBC) are found. The displacement of Western Pacific subtropical high (WPSH) is the main reason for the opposite distribution of extremes during EP and CP El Niño. In decay spring, the anticyclonic anomaly is found over WNP basin during EP El Niño. Southwest winds contribute to form the moisture source of extremes over SC (figure 1a). During CP El Niño, the anticyclonic anomaly extends westward to the east Bay of Bengal (EBOB), which impedes the moisture transported from the EBOB (figure 1b). In decay summer (Figure 1c and 1d), when EP (CP) El Niño occurs, the westerly jet (WJ) tends to be displaced southward (northward) in relation to the positioning of WPSH, contributing to stronger vertically integrated moisture flux convergence over SYR (MBC), while there is moisture flux divergence over MBC (SYR).
The different flow patterns associated with El Niño diversity appear to be forced by different SST warming signals in either tropical Indian Ocean or Maritime Continent regions, which can induce anomalous regional atmospheric circulation that affects the behaviors of WPSH and WJ.
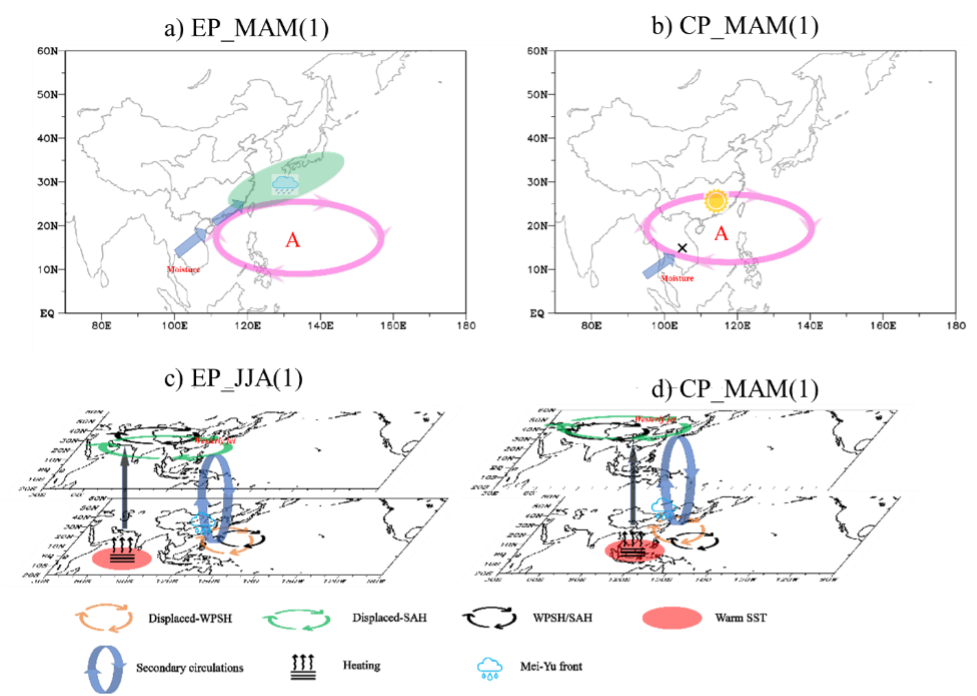
Figure 1. Mechanisms leading to extreme precipitation changes over SC in March-April-May (MAM), and SYR and MBC in June-July-August (JJA) during (a, c) EP and (b, d) CP EI Niño.
Evaluating CMIP model simulations of the Indian Ocean Dipole - the role of monsoon air-sea feedback on its skewness
Yi-Ling ZHENG
The Indian Ocean Dipole (IOD) is the dominant mode of interannual variability in the tropical Indian Ocean, characterized by surface cooling (warming) of the eastern Indian Ocean in its positive (negative) phase. Observed IOD events exhibit distinct amplitude asymmetry in relation to negative nonlinear dynamic heating (NDH) and asymmetric thermal damping. NDH is associated with the asymmetric nonlinear temperature advection and sea surface temperature (SST) response to thermocline changes. The thermal damping is caused by the cloud-radiation-SST feedback, which develops minor additional damping after cold SST anomaly leading to a cloud-free condition. In CMIP5, nearly all models simulate less-skewed IOD compared to the observed. On the other hand, for CMIP6, 6 out of 20 models show realistic skewness. Further examination reveals that the improvement of IOD skewness in CMIP6 over CMIP5 can be attributed to the former’s improved model mean states, particularly the western tropical Indian Ocean (WTIO) SST. Analyses of “less skewed models” indicate that when background SST in WTIO exceeds the SST for maximum precipitation sensitivity, the convective response to SST becomes asymmetric, leading to less zonal wind response and weaker zonal nonlinear advection during positive IOD. The warm SST bias in turn is associated with the weaker Indian summer monsoon. Additionally, ocean stratification also influences the IOD skewness: stronger stratification can lead to larger nonlinear SST response to thermocline changes; this is due to larger vertical temperature gradient resulting in larger nonlinear vertical advection. Our findings imply that substantial efforts should be made in reducing the Indian summer monsoon bias as well as advancing the upper-ocean stratification in the eastern Indian Ocean, for properly representing IOD in Earth System Models.
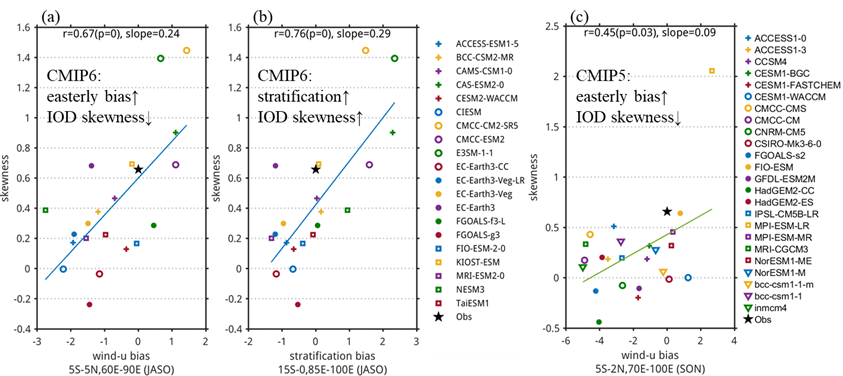
Figure 1. (a) Mean zonal wind bias versus IOD skewness in CMIP6. (b) Mean stratification bias versus IOD skewness in CMIP6. (c) Mean zonal wind bias versus IOD skewness in CMIP5.
The 2020 Summertime Extreme Marine Heatwave Events in the North Arabian Sea
Yungjia Lu
Marine heatwaves (MHWs) are defined as the prolonged warm sea surface temperature (SST) events that are above the climatological seasonally varying 90th percentile for at least five consecutive days, which can have a substantial negative impact on marine ecosystems and economies. In the boreal summer (June-August) of 2020, the North Arabian Sea experienced a record-breaking MHW event since 1982 with the duration of 84 days and the intensity of 1.9°C, followed by another comparably strong MHW event with the duration of 47 days in September and early October. Using satellite data and reanalysis products, we explored the relative contributions of different physical processes to these two consecutive MHW events by performing a mixed layer heat budget analysis.
The first extreme MHW was initiated by suppressed evaporation and surface wind, which were related to anomalous atmospheric circulation due to southwest tropical Indian Ocean warming. After its peak, enhanced total cloud cover and evaporative cooling led to the decay of this MHW event. However, in early September there was a revival of MHW in the same region, because of strengthened (suppressed) convection over southeastern part of the Arabian Sea (Northern Bay of Bengal) and low-level wind anomalies that were associated with the northward propagation of the boreal summer intraseasonal oscillation (BSISO) over northern Indian Ocean. It was found that, in additional to surface heat fluxes, mixed layer depth variation and ocean temperature advection were also important in shaping the MHW evolution. The roles of various climate modes, in particular the 2019 super Indian Ocean Dipole and the active BSISO over eastern Indian Ocean in 2020 were discussed.
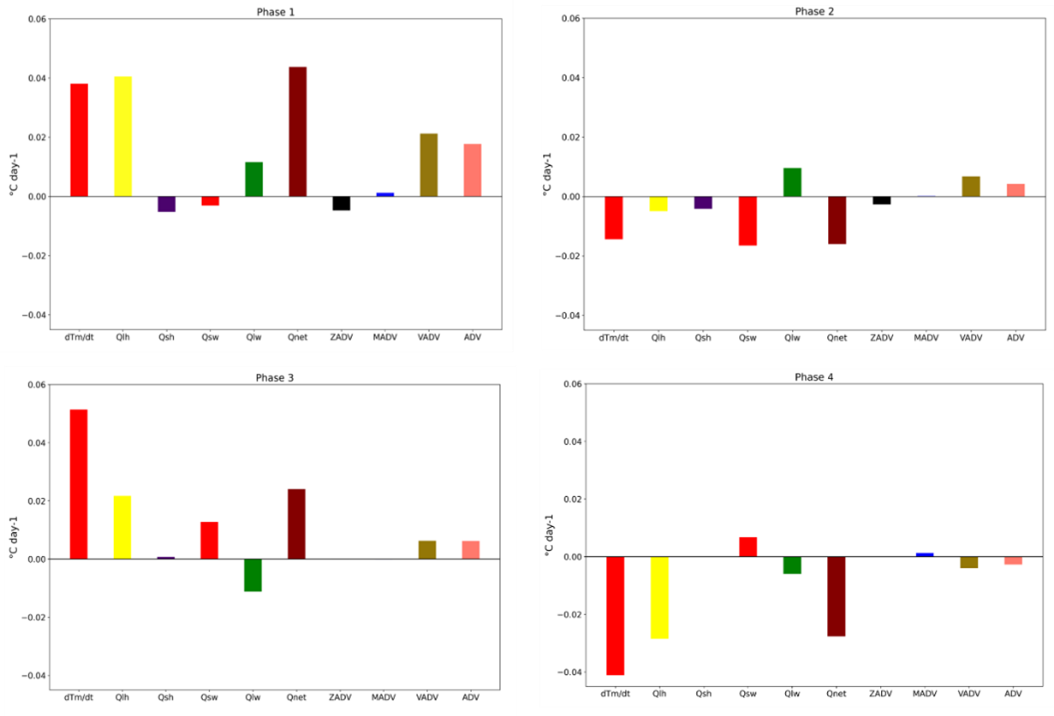
FIG 1. Different terms of mixed layer budget equation in four different phases.
Influence of anthropogenic warming on tropical cyclones activities
Tsun Ngai CHOW
Anthropogenic warming will alter the atmospheric and ocean environment, hence influencing tropical cyclone activities in the future. To a medium-to-high confidence that global tropical cyclone mean intensity in terms of lifetime maximum surface wind speed will have an increase by 5%, and differs among different ocean basins. Using high-resolution WRF model with a 15km and 3km nesting grid, future tropical cyclones intensity and structure due to thermodynamical changes in RCP8.5 and RCP4.5 emission scenario in the near and far future were simulated by pseudo-global warming technique. In the South China Sea Region, the intensities of the tropical cyclones in terms of maximum wind speed (Vmax) were simulated to have an increase under a warming environment. Moreover, the Radius of Maximum wind (RMW) of the simulated tropical cyclones was expected to have a decrease. Mean tropical cyclones’ size, defined as the radius of the gale-force wind (r17), were simulated to have minimal changes. However, the changes of Vmax, RMW and R17 among different tropical cyclones have a large variability. Relations of the changing sea surface temperature, vertical temperature profile, relative humidity and the vertical wind shear to the intensities and structures of the tropical cyclones were examined using multiple linear regression analysis.
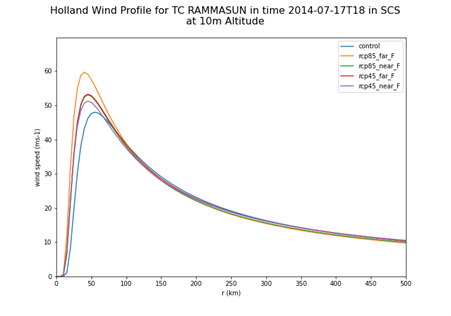
Azimuthally averaged surface wind profile fitted by Holland Model in near and far future under RCP8.5 and RCP4.5 emission scenario of Tropical Cyclone Rammasun (2014) during its peak intensity in the South China Sea region, indicating an increase in surface maximum wind speed and a decrease in the Radius of Maximum wind.
Island-Induced Eyewall Replacement in a Landfalling Tropical Cyclone: A Model Study of Super Typhoon Mangkhut (2018)
King Heng LAU
An unconventional, island-induced eyewall replacement (IER) occurred in Super Typhoon Mangkhut (2018) when it crossed Luzon Island. Upon landfall, its original compact eyewall broke down and dissipated rapidly. As Mangkhut exited Luzon and entered the South China Sea, a much larger new eyewall formed at a radius of 150–200 km from the storm center, three times larger than the original one. Unlike the eyewall replacement cycle in intense tropical cyclones, the breakdown of the original eyewall preceded the formation of the new eyewall (NEF) in Mangkhut. This evolution was reproduced reasonably well in a control experiment using the Weather Research and Forecasting Model. Two sensitivity experiments showed that the IER was triggered by Luzon Island, whose terrain is essential for not only the destruction of the original eyewall but also the NEF. In an axisymmetric framework, it is demonstrated for the first time that the NEF was preceded by the following processes: (1) an increase in the outward-directed agradient force in the boundary layer (BL) inflow region after landfall due to differential rates of weakening between the radial pressure gradient and the tangential wind, (2) creation of a BL deceleration zone, (3) localized reinforcement of BL inflow deceleration within the NEF region when Mangkhut re-entered the ocean, following the unbalanced dynamical pathway proposed by Y.-H. Huang et al. (2012), and (4) strengthening of the BL convergence and uplift which initiated and sustained the deep convection of the new eyewall.
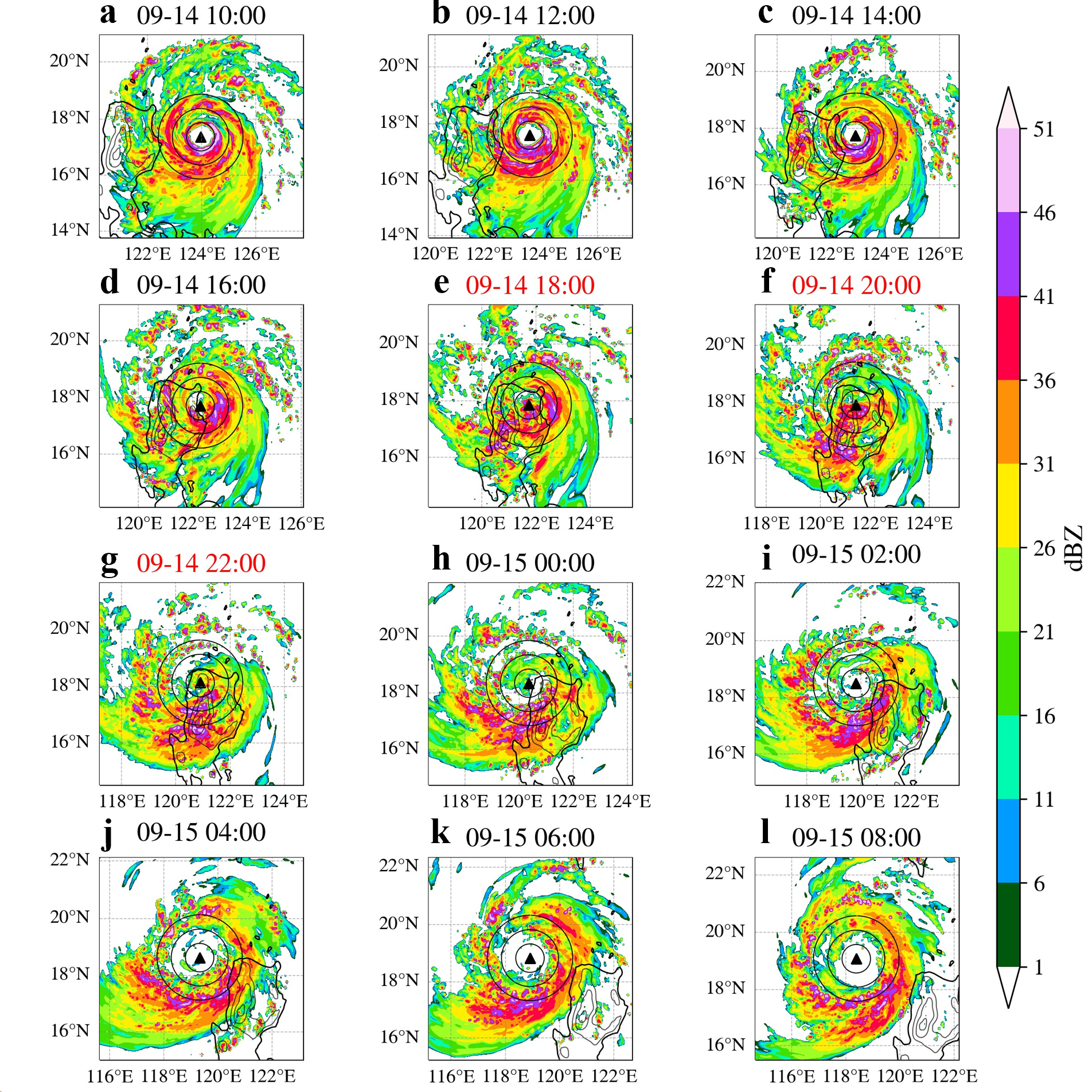
Fig 1. Simulated radar reflectivity (shading; units: dBZ) in CTL at 6 km altitude, above the freezing level. Timestamps are in UTC. Red timestamps indicate that the simulated TC center was over Luzon Island. The model terrain height of Luzon Island (in 500 m intervals) is indicated by black contours. Three circles at 55.5 km (0.5°), 111 km (1°), and 166.5 km (1.5°) from the storm center are plotted in each panel. The TC center automatically tracked by WRF is labeled by a black triangle.
[1] Lau, K. H., Tam, C.-Y., & Wu, C.-C. (2024). Island-Induced Eyewall Replacement in a Landfalling Tropical Cyclone: A Model Study of Super Typhoon Mangkhut (2018). Journal of Geophysical Research: Atmospheres, Accepted.
[2] Huang, Y.-H., Montgomery, M. T., & Wu, C.-C. (2012). Concentric Eyewall Formation in Typhoon Sinlaku (2008). Part II: Axisymmetric Dynamical Processes. Journal of the Atmospheric Sciences, 69(2), 662-674.